Ecosystem Services of Habitat-Friendly Solar Energy
Leroy J. Walston, Heidi Hartmann, Laura Fox, Michael Ricketts, Ben Campbell, and Indraneel Bhandari, Argonne National Laboratory
This section highlights several types of agrivoltaic options related to ecosystem services that include siting considerations, ecological impacts of dual-use sites, construction methods and habitat restoration strategies. One type focuses on ecologically focused siting, construction, and vegetation management principles in an effort to make photovoltaic (PV) solar energy more ecologically compatible. This includes minimizing ecological impacts associated with siting and construction and improving the ecological value of the site through habitat enhancement. Given its ecological focus, this form of agrivoltaics design is often referred to as ecovoltaics (Sturchio and Knapp, 2023; Tölgyesi et al., 2023).
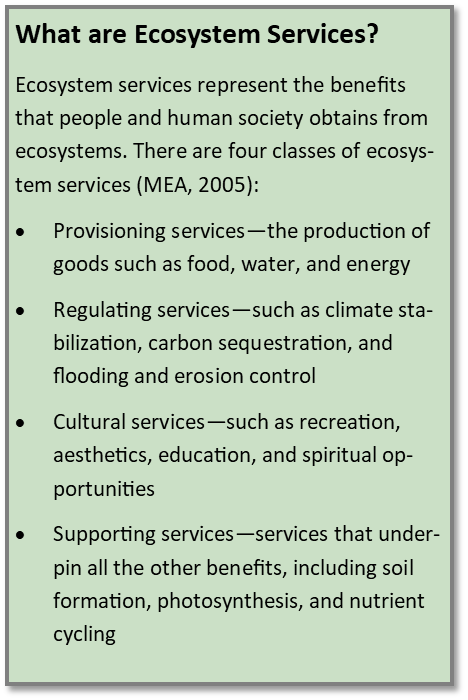
The co-location of solar energy and habitat restoration (i.e., habitat-friendly solar‘ or solar-pollinator habitat) has become the most popular ecovoltaics strategy to safeguard biodiversity and improve the site’s ecosystem services output. Habitat-friendly solar designs typically focus on the planting and establishment of deep-rooted and regionally appropriate native grasses, wildflowers, and other non-invasive naturalized flowering plant species. The habitat created at these sites could support insect pollinators and other wildlife and improve other ecosystem services of the site (Figure 1).
But what ecosystem service benefits might be realized at solar facilities managed for habitat? Agrivoltaics can broadly improve the output of all classes of ecosystem services (Figure 2). Conceptually, solar-pollinator habitat has the potential to improve the outputs of all classes of ecosystem services (Table 1).
The pairing of solar energy and habitat enhancement sounds like a logical win-win for clean energy and biodiversity. However, several factors can influence the feasibility and ecological effectiveness of solar-pollinator habitat, such as geography, seed availability and cost, previous land use, soil type, and solar size and design (e.g., PV panel height and spacing). Several scientific studies have been conducted in recent years to examine different solar-pollinator habitat configurations and management options. Two studies in particular are the Innovative Solar Practices Integrated with Rural Economies and Ecosystems (InSPIRE; openei.org/wiki/InSPIREopenei.org/wiki/InSPIRE) and Pollinator Habitat Aligned with Solar Energy (PHASE; rightofway.erc.uic.edu/phase). Both projects are funded by the U.S. Department of Energy (DOE) Solar Energy Technologies Office (SETO) and include a focus on the ecological and economic implications of solar-pollinator habitat. Results from these studies have shed light on which vegetation establishes at solar sites based on their unique management needs and the amount of time required for vegetation to establish and for biodiversity responses to be measured. These studies incorporate the research findings intoguidelines and toolkits to assist the site-specific selection of seed mixes and management strategies to optimize the performance of solar-pollinator habitat based on ecological and economic (budget) objectives.

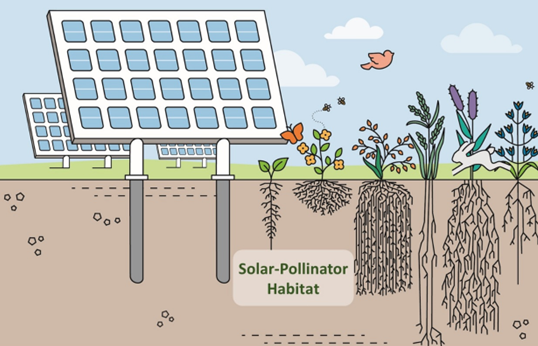

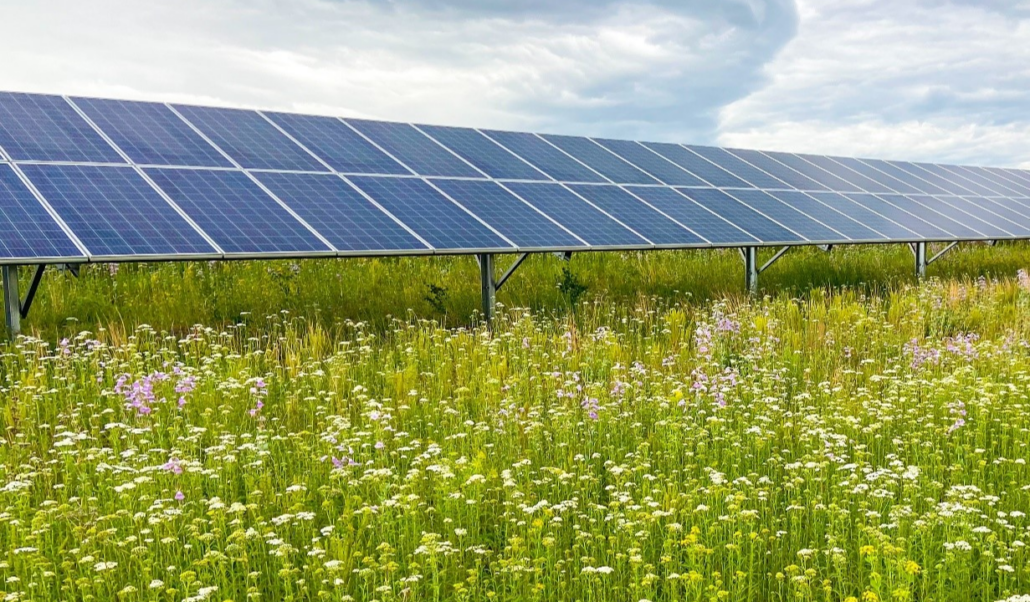
Figure 1. A) Illustration of the theoretical ecosystem services of solar-pollinator habitat. Compared to conventional groundcover, such as turfgrass, solar-pollinator habitat can provide higher-quality habitat for biodiversity. B) Example image of solar-pollinator habitat at a solar site in Minnesota. Images: Argonne National Laboratory
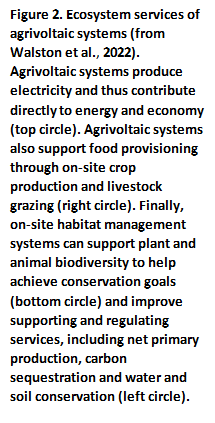
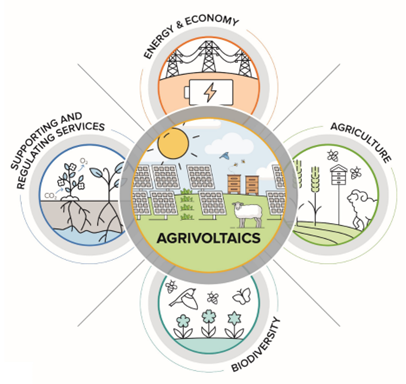
Table 1. Potential Ecosystem Services of Solar-Pollinator Habitat.
Ecosystem Service | Benefit |
Biodiversity conservation (broadly linked to all ecosystem service classes) | Solar-pollinator habitat can safeguard biodiversity by supporting a larger diversity of organisms and communities. This could benefit several ecosystem services, such as food production (provisioning), recreation (cultural), water conservation (regulating), and nutrient cycling (supporting) (Walston et al., 2021, 2022, 2024; Blaydes et al., 2024). |
Energy production (provisioning service) | Solar-pollinator vegetation can create favorable microclimates to improve PV panel performance (Choi et al., 2023). |
Food production (provisioning service) | Solar-pollinator habitat can improve populations of insect pollinators and predators, which can benefit nearby agricultural production (Walston et al., 2024). |
Carbon sequestration and soil health (regulating services) | The establishment of solar-pollinator habitat typically involves soil and vegetation management practices that allow for greater soil carbon sequestration over time, compared to other land uses (Walston et al., 2021). |
Stormwater and erosion control (regulating service) | Deep-rooted solar-pollinator habitat can help stabilize soil and minimize runoff (Walston et al., 2021). |
Nutrient cycling and air quality (supporting services) | Solar-pollinator habitat can improve nutrient cycling and air quality (Wratten et al. 2012; Agostini et al., 2021). |
Aesthetics and recreation (cultural services) | Solar-pollinator habitat can improve human perception public acceptance of the solar site (Moore et al., 2021). |
What are best practices for establishing solar-pollinator habitat?
There is growing science-based evidence on the ecological effectiveness of solar-pollinator habitat. Most of this research focuses on two main aspects: 1) vegetation establishment and management; and 2) biodiversity responses (Figure 2). One critical need for the solar industry has been assistance in selecting the seed mix design and vegetation management tools that would optimize the establishment of solar-pollinator habitat for a site’s specific physical characteristics (e.g., geographic region, soil type), PV site design (e.g., plant height restrictions), and budget. To help guide these decisions, the DOE PHASE project has produced a series of tools to inform solar-pollinator habitat planting implementation, seed selection, cost comparisons, and habitat assessment (Figure 3).
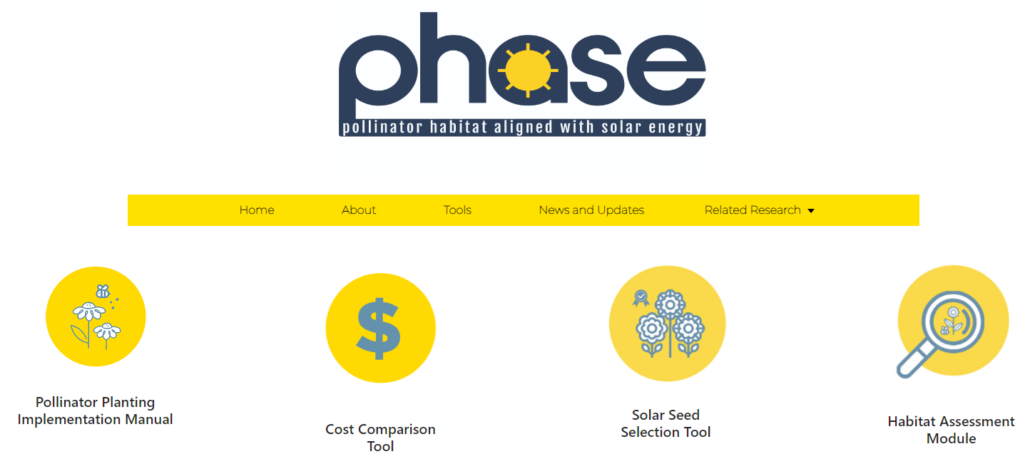
Figure 3. Solar-pollinator habitat decision support toolkits developed through the DOE PHASE project. Source: rightofway.erc.uic.edu/phase-toolkits/rightofway.erc.uic.edu/phase-toolkits/.
What do we know about the effectiveness of solar-pollinator habitat?
This section highlights objectives and outcomes from field research projects funded by DOE to understand the ecosystem services of solar-pollinator habitat. Two case studies are presented: 1) potential biodiversity benefits of solar-pollinator habitat; and 2) potential benefits of solar-pollinator habitat for soil health.
Case Study 1: If You Build It, They Will Come
A recent study from the DOE InSPIRE project examined the biodiversity responses for five years following the establishment of solar-pollinator habitat (Walston et al., 2024). The research was conducted at two Minnesota PV solar facilities owned and operated by Enel Green Power. The research team from Argonne National Laboratory, National Renewable Energy Laboratory, and Minnesota Native Landscapes conducted a longitudinal field study over five years (2018 to 2022) to understand how insect communities responded to newly established habitat on solar energy facilities in agricultural landscapes. Specifically, they investigated: 1) temporal changes in flowering plant abundance and diversity; 2) temporal changes in insect abundance and diversity; and 3) pollination services of solar-pollinator habitat to nearby agricultural fields. The team found increases over time for all habitat and biodiversity metrics. For example, by 2022, the researchers observed a sevenfold increase in flowering plant species richness, and native abundance increased by over 20 times the numbers initially observed in 2018 (Figure 4). The research team also found positive effects of proximity to solar-pollinator habitat on bee visitation to nearby soybean (Glycine max) fields. Bee visitation to soybean flowers adjacent to solar-pollinator habitat were greater than bee visitation to soybean field interior and roadside soybean flowers (Figure 5). These observations highlight the relatively rapid (less than four years) insect community responses to solar-pollinator habitat. This study also demonstrates that, if properly sited and managed, solar-pollinator habitat can be a feasible way to safeguard biodiversity and increase food security in agricultural landscapes. Photos of solar-pollinator habitat insects visiting the on-site vegetation at these sites are shown in Figure 6.


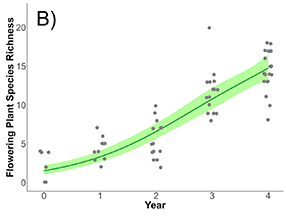
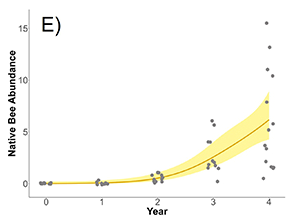
Figure 4. Observed and predicted measures of (A) flowering plant species richness and (B) native bee abundance recorded over time at two PV solar facilities planted with pollinator-friendly habitat in Minnesota. (Walston et al., 2024).
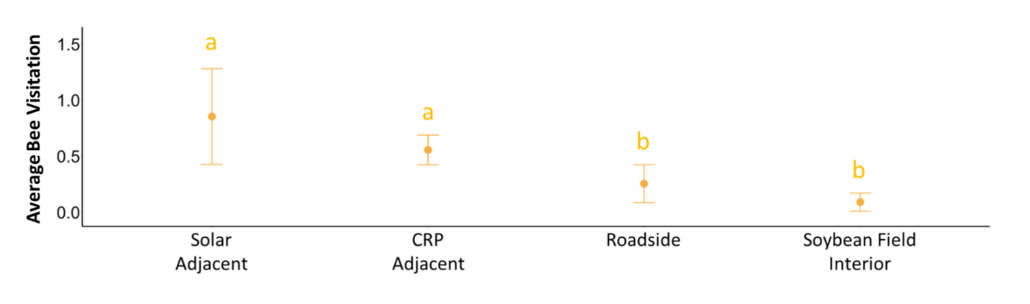
Figure 5. Observed bee visitation to soybean flowers at different field locations in Minnesota. Different letters indicate statistically different groups at the p = 0.05 level (Walston et al., 2024).
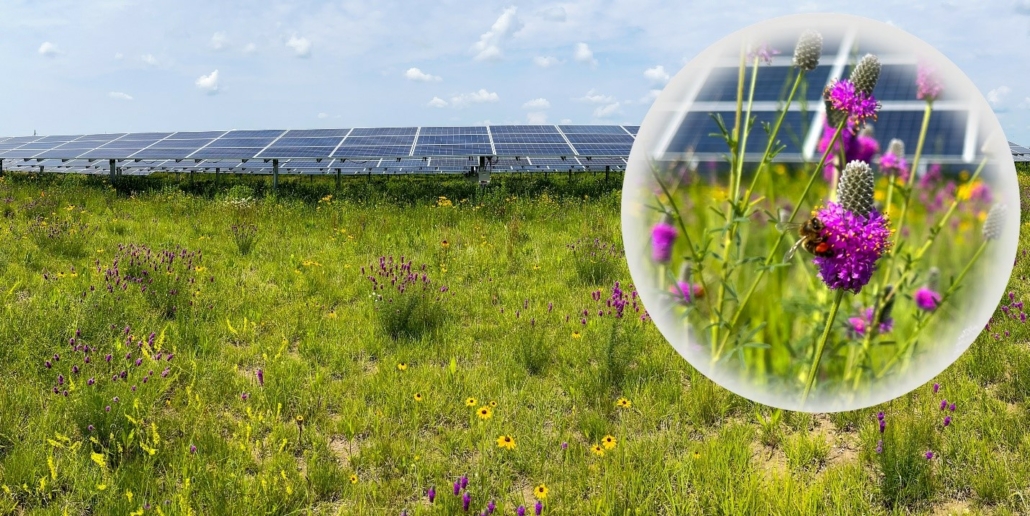
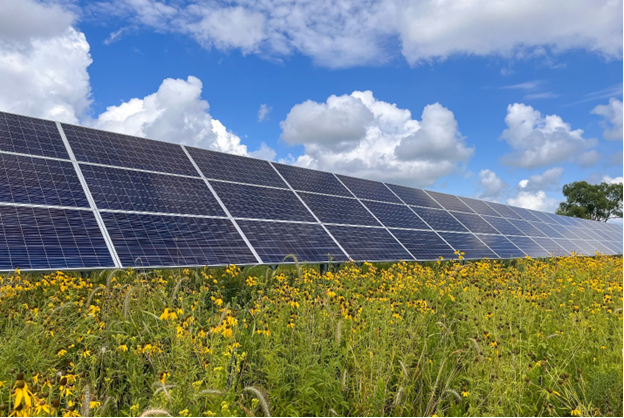
Figure 6. Solar-pollinator habitat and insects observed at solar facilities in Minnesota. Top: solar-pollinator habitat dominated by purple prairie clover and black-eyed Susan flowers, with a honeybee visiting a flower (inset). Bottom: solar-pollinator habitat dominated by yellow coneflower. Photos: Argonne National Laboratory
Case Study 2: Soil Health Benefits of Solar-Pollinator Habitat
As PV solar energy sites become increasingly common, there is growing interest in identifying potential co-benefits, in addition to energy production, that could be provided using the same land area (Choi et al., 2023). These co-benefits include a variety of both economic and ecosystem services, many of which rely greatly on preserving, restoring, and/or maintaining a healthy soil environment, which is itself a valuable ecosystem service. Healthy soils are key to supporting and nurturing plant growth, and solar facilities offer a unique opportunity to improve soils that are either naturally low-quality or have been degraded from decades of agriculture. This can be accomplished through a variety of strategic planning initiatives and land management practices that focus on minimizing soil and vegetation disturbances and encouraging the establishment of ecologically friendly and sustainable ecosystems. By understanding the relationships and interactions that exist between plants and the soil environment, we can gain valuable insights into how to maximize land-use efficiency and increase sustainable land management practices over the large areas of land that will be required for utility-scale solar facility development needed to achieve the renewable energy goals of the United States by 2050.
Just as healthy soil is necessary to support plant growth, plants can help improve soil health through various mechanisms (Figure 7). Soil health is characterized by a combination of physical, chemical, and biological properties, including bulk soil density, water infiltration and holding capacity, soil organic carbon and available nutrient contents, soil pH and cation exchange capacity, and microbial activity and diversity. Plant roots, especially those from deep-rooting perennial species (such as are found in many pollinator seed mixes), help reduce soil erosion and improve soil structure by providing a supportive network of course and fine roots that stabilize soil particles and aggregates while simultaneously improving water infiltration. Plants also supply organic matter, carbon, and other nutrients to the soil environment viasurface leaf litter, root exudates, and root litter. These organic matter inputs serve as nutrient pools for micro- and macro-organisms in the soil, and to increase soil water-holding capacity. Additionally, a portion of the carbon from plant organic matter inputs and microbial necromass will end up becoming associated with soil minerals to form mineral-associated organic matter (MAOM), which can have very long residence times in soil and serve as a carbon sink for atmospheric CO2 (Bai and Cotrufo, 2022).
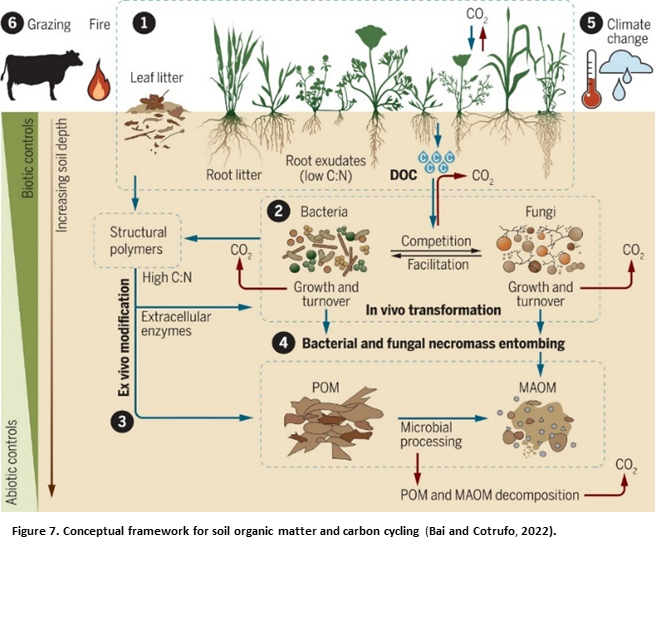
There are many ways that vegetation can be used at solar facility sites to provide additional benefits beyond increasing soil health. While there is much research that has shown the positive effects of vegetation on soil health, research that specifically addresses how soil health indicators are affected by land management practices at solar facilities is lacking. Given what is known, it is reasonable to expect that sustainable vegetation management at solar facilities will result in improved soil health over time. However, this is likely dependent on the degree of disturbance sustained during site construction, and possibly any number of other controlling factors, such as local climate, native vegetation, and/or soil type. For example, Choi et al. (2020) found that even after seven years of revegetation at a solar facility site in Colorado, carbon and nitrogen concentrations had not recovered to comparable levels of adjacent reference grasslands. The authors attributed this to the significant amount of topsoil removal and grading that occurred during site construction, which significantly disturbed and mixed the soil profile, resulting in severely reduced surface carbon and nitrogen levels. However, this study did not compare vegetated areas to non-vegetated areas within the site. Another study by Choi et al. (2023) did make this comparison at a site in Minnesota where topsoil removal and grading were avoided. The researchers found that revegetated areas had significantly more carbon, nitrogen, and other nutrients levels relative to the areas that were left bare and were ultimately similar to adjacent control plots (Figure 8). This disparity in results and lack of clear data presents a challenge to understanding soil health dynamics as it relates to land management practices at solar facilities.
Fortunately, DOE SETO has sponsored a project whose sole focus is to gather soil data from solar facilities across a wide range of environments in the United States that can hopefully address this question. This project, Ground-mounted Solar and Soil Ecosystem Services, is being led by Argonne National Laboratory and will provide standardized guidance on measuring and analyzing soil parameters central to soil health at solar facilities, and establish a national database of solar facility soil data that will hopefully shed light on how vegetation and land management at solar facilities can impact soil health over time.
REFERENCES
Agostini, A., M. Colauzzi, and S. Amaducci. 2021. Innovative agrivoltaic systems to produce sustainable energy: an economic and environmental assessment. Applied Energy. 281: 116102.
Bai, Y. and M.F. Cotrufo. 2022. Grassland soil carbon sequestration: Current understanding, challenges, and solutions. Science. 377: 603–608.
Blaydes, H., S.G. Potts, J.D. Whyatt, and A. Armstrong. 2024. On-site floral resources and surrounding landscape characteristics impact pollinator biodiversity at solar parks. Ecological Solutions and Evidence. 5: e12307.
Choi, C.S., A.E. Cagle, J. Macknick, D.E. Bloom, J.S. Caplan, and S. Ravi. 2020. Effects of Revegetation on Soil Physical and Chemical Properties in Solar Photovoltaic Infrastructure. Frontiers in Environmental Science. 8: 140.
Choi, C.S., J. Macknick, Y. Li, D. Bloom, J. McCall, and S. Ravi. 2023. Environmental Co‐Benefits of Maintaining Native Vegetation with Solar Photovoltaic Infrastructure. Earth’s Future. 11: e2023EF003542.
Millenium Ecosystem Assessment (MEA). 2005. Ecosystems and Human Well-Being: Synthesis. Island Press, Washington, DC.
Moore, S., H. Graff, C. Ouellet, S. Leslie, D. Olweean, and A. Wycoff. 2021. Developing Utility-Scale Solar Power in Michigan at the Agriculture-Energy Nexus. Stakeholder Perspectives, Pollinator Habitat, and Trade-offs. Report for the Institute for Public Policy and Social Research, Michigan State University. Available at ippsr.msu.edu/mappr/developing-utility-scale-solar-power-michigan-agriculture-energy-nexus. Accessed March 29, 2024.
Sturchio, M.A. and A.K. Knapp. 2023. Ecovoltaic principles for a more sustainable, ecologically informed solar energy future. Nature Ecology & Evolution. 7: 1746-1749.
Tölgyesi, C., Z. Bátori, J. Pascarella, et al. 2023. Ecovoltaics: framework and future research directions to reconcile land-based solar power development with ecosystem conservation. Biological Conservation. 285: 110242.
Walston, L.J., Y. Li, H.M. Hartmann, J. Macknick, A. Hanson, C. Nootenboom, E. Lonsdorf, and J. Hellmann. 2021. Modeling the ecosystem services of native vegetation management practices at solar energy facilities in the Midwestern United States. Ecosystem Services. 47: 101227.
Walston, L.J., T. Barley, I. Bhandari, B. Campbell, J. McCall, H.M. Hartmann, and A.G. Dolezal. 2022. Opportunities for agrivoltaic systems to achieve synergistic food-energy-environmental needs and address sustainability goals. Frontiers in Sustainable Food Systems. 16: 932018.
Walston, L.J., H.M. Hartmann, L. Fox, J. Macknick, J. McCall, J. Janski, and L. Jenkins. 2024. If you build it, will they come? Insect community responses to habitat establishment at solar energy facilities in Minnesota, USA. Environmental Research Letters. 19: 014053.
Wratten, S.D., M. Gillespie, A. Decourtye, E. Mader, and N. Desneux. 2012. Pollinator habitat enhancement: benefits to other ecosystem services. Agriculture, Ecosystems & Environment. 159: 112-122.