Tag Archive for: AgriSolar
Across the country, farmers, landowners, researchers, and solar companies are working together to harvest the sun twice: once with crops, honey, pollinators, and forage for grazing animals, and again with solar panels. This co-location of solar and agriculture is known as agrisolar or agrivoltaics. In Harvesting the Sun, the leading voices of the agrivoltaic movement come together to share their stories and shine a light on a climate solution that can increase farm profitability, save valuable water, improve the soil, provide shade for farm workers, develop valuable ecosystem services, and increase the resiliency of rural communities.
If you would like to contribute to NCAT’s development of an Agrisolar Center to continue this work, contact us at agrisolar@ncat.org.
By Savannah Crichton, University of Alaska Fairbanks
Southcentral Alaska is home to the state’s first agrivoltaics project, a study that aims to uncover the best practices for harvesting from both land and sun. The research team will monitor both farmed crops and native berry plants that grow between the rows of panels at an operational solar PV array. The solar array is situated in the Matanuska-Susitna Valley, where the majority of Alaska’s farmland is located.
The project, Agrivoltaics: Unlocking Mid-Market Solar in Rural Northern Climates, is a three-year project funded by the U.S. Department of Energy (DOE) Solar Energy Technologies Office (SETO).
In 2023, solar developer and project partner Renewable IPP (RIPP) built an 8.5-megawatt solar array in Houston, Alaska, which was financed by diversified clean energy company, CleanCapital. This array is classified as mid-market solar–the middle ground between commercial solar projects and large-scale (>100MW+) utility solar. RIPP sells the electricity produced at this site to Matanuska Electric Association, the regional utility.
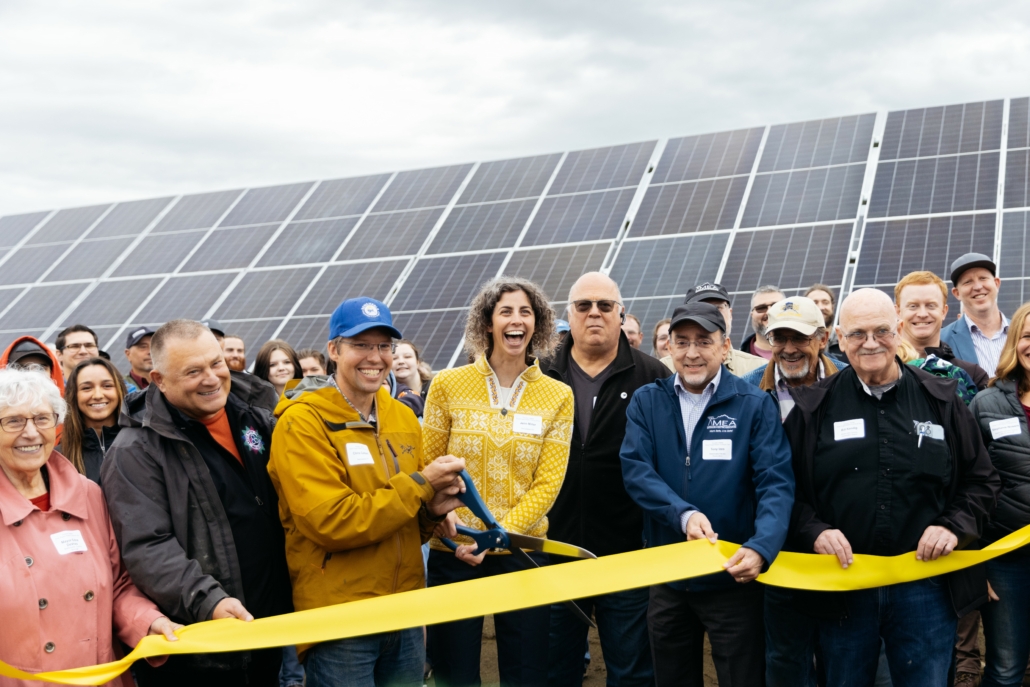
Ribbon cutting at the solar array in Houston, Alaska.
At northern latitudes, the sun hits the earth at a lower angle, causing solar panels to shade each other during sunrises and sunsets. To maximize energy production and avoid shading, solar developers may increase row spacing. With intentional design and wider rows, there’s ample land open between these rows for foraging or farming.
The new Houston array is situated on a berry stand well known to local berry pickers. Drawing from their previous solar farm development experience, RIPP intentionally found a way to minimize the environmental impact of solar construction and increase community acceptance by maintaining as much of the native vegetation as possible. Some of the boreal species growing onsite include willow, alder, birch, moss, fireweed, labrador tea, bog blueberry, and lingonberry. The latter two are edible berry species that carry meaningful value to Alaska Native cultures and are prized by many in Alaska’s summer months.
Instead of aggressive clearing methods that level land and remove certain ecological services, a low-mulching protocol was used to preserve topsoil and low-growing woody shrubs. Native low-growing species, like berries, can continue to grow and sequester carbon. If nutrient-rich soil is left intact, solar developers leave options open for the development of agrivoltaic applications to co-locate their array with food production.
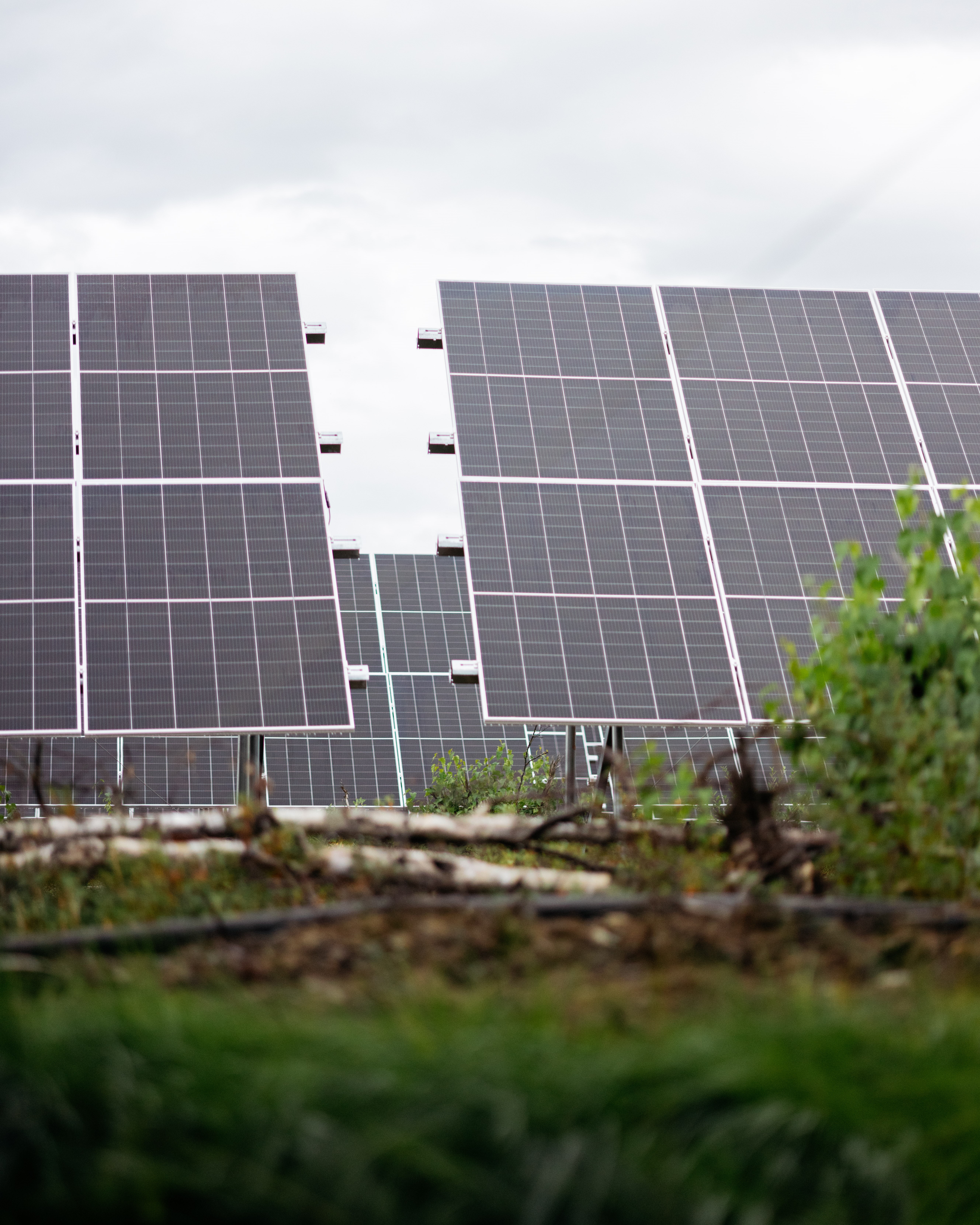
Blueberry bushes growing on the solar site.
That’s exactly what a team of researchers at UAF from the Alaska Center for Energy and Power (ACEP) and the Institute of Agriculture, Natural Resources and Extension (IANRE) intend to study. UAF is one of six projects funded under the Foundational Agrivoltaic Research for Megawatt Scale (FARMS) program to conduct research on agrivoltaic opportunity for their communities.
Principal Investigator Christopher Pike from ACEP and co-investigators Glenna Gannon and Jessie Young-Robertson pulled together an interdisciplinary team of engineers, farmers, and solar experts. The research team is joined by Alaska Pacific University (APU) Spring Creek Farm Manager and project co-investigator Benjamin Swimm,and RIPP founders Jenn Miller and Chris Colbert.
Under the mission to bolster food and energy security, the team will measure both solar PV production and physiological health of crops over two growing seasons, develop a techno-economic analysis to guide future mid-market solar PV and agriculture projects, and connect with the community through educational programming.
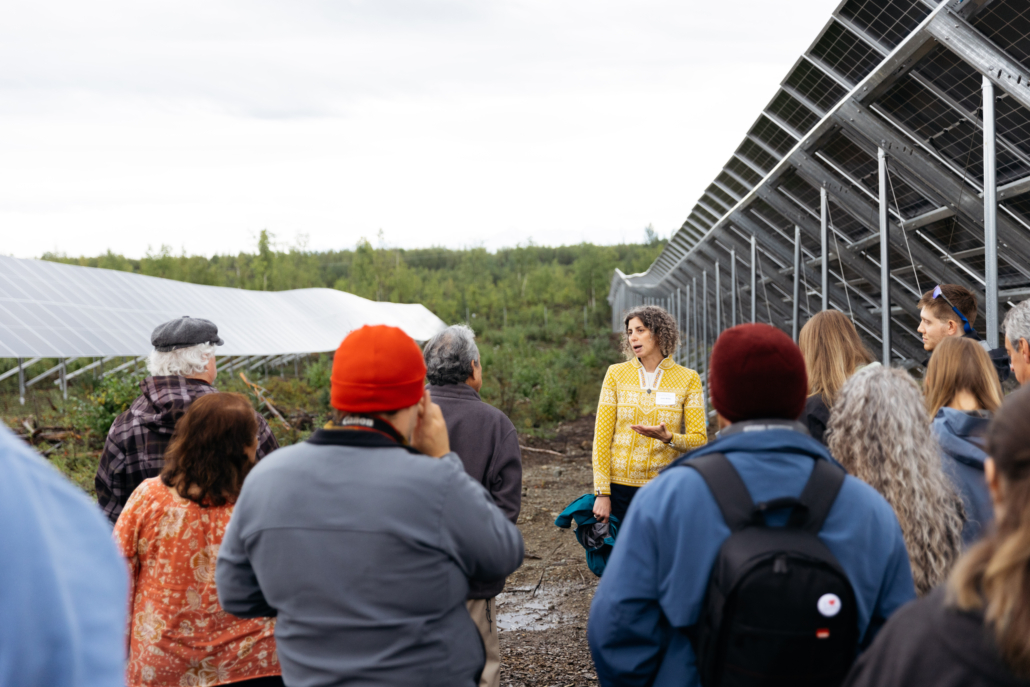
CEO and Manager of Renewable IPP Jenn Miller speaks to crowd at the solar farm.
In the first few months of the project, the team compiled a diverse stakeholder pool of northern and Alaska-based landowners, farmers, utilities, solar developers, tribal organizations, academic researchers, and environmental agencies. Through individual outreach, team networks, and local events, over 200 people signed up to participate in a stakeholder needs assessment survey.
The survey was distributed to evaluate stakeholder perspectives towards agrivoltaics in rural northern contexts. In addition to this data, the team will conduct a techno-economic analysis to understand the economic conditions in Alaska that may create hurdles or opportunities for those interested in developing agrivoltaic systems.
The contributions from the stakeholder survey and follow-up interviews will inform the project’s agricultural research plans and economic analysis. Broadly, this input helps the team understand community acceptance and potential adoption of multi-use solar farms while also adding color to the picture of food and energy security in rural, northern regions.
Preparation of the agricultural research plots at the Houston array will begin in summer 2024. The acidic silt loam soil will be amended with lime and compost in plot locations to make them more amenable to agricultural growth.
With the soils tilled, planting will begin in summer 2025. A combination of popular commercial vegetables and animal forage crops will be planted and monitored throughout the growing season for their productivity both inside and outside the solar array.
Crops at northern latitudes undergo unique challenges, like cool growing seasons and high solar radiation loads. Because of these unique conditions, some crops grown under solar PV arrays may experience improved productivity, while other crops that are usually productive in the rural north may not perform as well.
Gannon, Young-Robertson, and ACEP research professional Savannah Crichton will coordinate the collection of plant physiology data of the agricultural crops, as well as the existing blueberry and lingonberry plants. Leaf-level physiological measurements of photosynthesis, transpiration, water use, and stress help define the dimensions of health in plants. These measurements will allow the team to understand the impact that fixed solar modules and increased shade have on the plants’ overall health, crop yield, and produce quality.
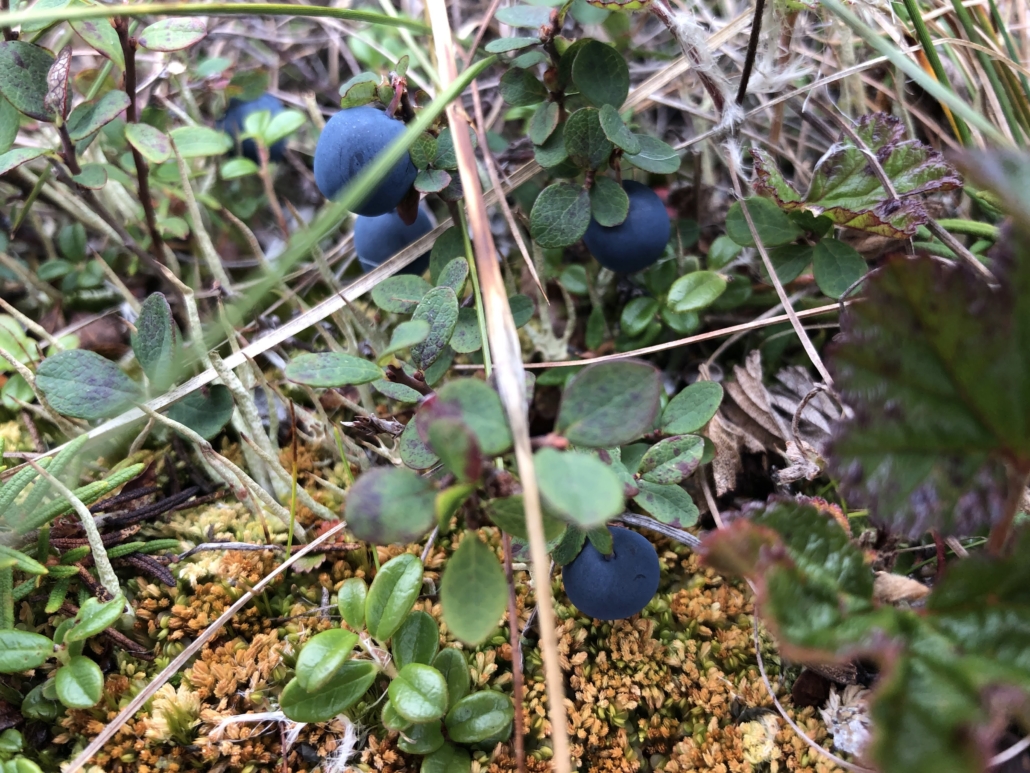
Close-up of the blueberries growing on-site.
Likewise, Pike and ACEP research engineer Henry Toal will monitor the solar power production and gauge the impact of farming activities on the array’s operation and maintenance costs. Weaving together qualitative, economic, physiological, and electrical data will allow the team to evaluate the feasibility of agrivoltaic systems in the north.
Rural households in Alaska spend nearly 27% of their annual income on energy expenses, and around 95% of Alaska’s non-subsistence food supply is imported. If an agrivoltaic model works in Alaska, it could be a major breakthrough for increasing food and energy security in the state. The impacts of this research have significant potential value, not just for solar developers and farmers, but for entire communities.
If you’d like to learn more about the project, visit our website or email Savannah at sgcrichton@alaska.edu.
The research is based upon work supported by the U.S. Department of Energy’s Office of Energy Efficiency and Renewable Energy (EERE) under the Solar Energy Technologies Office (SETO) Award # DE-EE0010442.
Farmers Across America Chase Stable Returns
“Farmers are increasingly embracing solar as a buffer against volatile crop prices and rising expenses. Their incomes are heading for a 26% slide this year, the biggest drop since 2006, as cash receipts for corn, soy and sugar cane are expected to drop by double-digit percentages.
The shift is a big part of the renewables push in the US: The American Farmland Trust estimates that 83% of expected future solar development will take place on agricultural soil.” – bloomberg.com
Agrisolar Market Forecast to be Worth $10.64 Billion by 2033
“The Brainy Insights estimates that the USD 2.98 billion Agrivoltaics market will reach USD 10.64 billion by 2033. Increased government initiatives to boost R&D in agrivoltaics is one major factor that may create lucrative opportunities for agrivoltaics devices in the market. Governments across the globe have undergone tremendous initiatives to boost investments and increase subsidies in the market. To achieve net-zero carbon emissions the government across the globe is undergoing a renewable fuel-based economy.” – finance.yahoo.com
US Farms with Solar Have Tripled Since 2013
“Solar panels are gaining popularity across U.S. fields. In fact, there are now three times as many farms with solar installations compared to 2012.
In 2012, a little more than 36,000 U.S. farms had them installed. By 2017, that number had jumped to more than 90,000. In 2022, it shot up to nearly 120,000.
Successful Farming found some producers were being offered as much as $1,000 per acre to lease land for solar. While crops can net that much, the panels do not require any input costs like seed and fertilizer.” – rfdtv.com
In this explainer video, AgriSolar Clearinghouse Director Stacie Peterson offers up five things to know about agrisolar and crops.
In this explainer video, AgriSolar Clearinghouse Director Stacie Peterson offers up five things to know about agrisolar and grazing.
In this explainer video, AgriSolar Clearinghouse Director Stacie Peterson offers up five things to know about agrisolar and pollinator habitat and beekeeping.
his work simulates the behavior of solar irradiance and its interaction with photovoltaic panels and the crop, as well as possible shading, in a photovoltaic plant to study its potential reconversion into an agrivoltaic installation.
This study aims to compare two agrivoltaic systems (stilted and vertical bifacial) from cradle-to-gate with the life cycle assessment method using a system expansion approach. Further, an unmodified agricultural production and total substitution of the latter by photovoltaic-modules (photovoltaic-scenario) are assessed.
This study investigates the use of a foldable solar panel system equipped with a dynamic tracking algorithm for agrivoltaics system (AVS) applications. It aims to simultaneously meet the requirements for renewable energy and sustainable agriculture.